Advanced Microscopy and Spectroscopy for Probing and Optimizing Anionic Redox in High Energy Lithium Batteries
The earliest developed LiCoO2 layered oxide cathode material sparked the development of other layered cathode materials, dominating the positive electrode materials for lithium ion batteries. Within the practical operating conditions of today, the current generation layered oxide materials do not meet the future energy storage demands of 350 Wh kg-1 per cell. This roughly translates to over 800 Wh kg-1 at the positive electrode level. Li-excess materials have the potential to meet the high energy demands. Unlike the classical layered oxides, Li-excess materials exhibit capacities that go beyond conventional topotactic mechanistic theoretical values because of reversible and stable anionic redox.
In the past five years, our research group has made great progress on developing advanced characterization techniques (including coherent X-ray imaging, neutron pair distribution function, and resonant inelastic X-ray scattering) coupled with atomic scale modeling to properly characterize the dynamic phenomena that govern the anionic redox related performance limitations of Li-excess materials. Furthermore, our efforts have improved the material synthesis and surface modification to improve capacity retention. It is through the in-depth understanding of these anionic redox based cathode materials at atomistic and molecular level and their dynamic changes during the operation of batteries; we can successfully formulate strategies to optimize this class of cathode materials.
Highlighted Publications:
1. M. Zhang, B. Qiu, J. M. Gallardo-Amores, M. Olguin, H. Liu, Y. Li, C. Yin, S. Jiang, W. Yao, M. Elena Arroyo-de Dompablo, Z. Liu and Y. S. Meng, “High Pressure Effect on Structural and Electrochemical Properties of Anionic Redox- Based Lithium Transition Metal Oxides“, Matter, 2020, ASAP
2. A. Singer, M. Zhang, S. Hy, D. Cela, C. Fang, T. A. Wynn, B. Qiu, Y. Xia, Z. Liu, A. Ulvestad, N. Hua, J. Wingert, H. Liu, M. Sprung, A. V. Zozulya, E. Maxey, R. Harder, Y. S. Meng, and O. G. Shpyrko,”Nucleation of Dislocations and Their Dynamics in Layered Oxide Cathode Materials During Battery Charging‘ ,Nature Energy, 2018, 3, 641
3. B. Qiu, M. Zhang, L. Wu, J. Wang, Y. Xia, D. Qian, H.D. Liu, S. Hy, Y. Chen, K. An, Y. Zhu, Z. Liu, Y. S. Meng, “Gas–solid interfacial modification of oxygen activity in layered oxide cathodes for lithium-ion batteries“, Nature Communication 2016, 7,12108
4. Sunny Hy, H.D. Liu, M. Zhang, D. Qian, B.-J. Hwang, Y. S. Meng, “Performance and design considerations for lithium excess layered oxide positive electrode materials for lithium ion batteries“, Energy & Environmental Science, 2016, 9, 1931
Co Free Cathode Materials and Their Novel Architectures
The next generation Li-ion batteries (LIBs) need reasonable matching of electrode and electrolyte to achieve the best performance, including longer cycling and better safety. For the cathode materials, the current commercialization and research mainly focuses on lithium metal oxides (LiNixCoyMnzO2, x+y+z=1) with layered structure. Layered lithium metal oxides have high energy densities, but the use of expensive and toxic cobalt elements greatly restricts their application in widespread commercialization of electric vehicles. In this context, a new spinel type oxide LiNi0.5Mn1.5O4 (LNMO) is of great focus with a theoretical capacity of 147 mAh/g and average working voltage as high as 4.7 V. More importantly, it does not contain expensive cobalt, which makes LNMO cathode cost-effective and suitable for applications in the field of power batteries and large-scale energy storage.
The objective of this project is to research, develop, and demonstrate a spinel type LNMO electrode and novel electrolyte formulation for use in next-generation LIBs. Our proposed cathode is 100% free of cobalt and its novel architecture will have porosity less than 20% and designed tortuosity for high rate capability. In addition, to guide our research to determine which electrolyte system is more stable and compatible for LNMO electrode materials under high voltage cycling, we will develop a series of characterization techniques such as ex-situ X-ray photoelectron spectroscopy (XPS), ex-situ cryogenic transmission electron microscopy (cryo-TEM), ex-situ cryogenic focused ion beam microscope (cryo-FIB) and in situ time-of-flight secondary-ion mass spectrometry (TOF-SIMS).
Highlighted Publications:
1. W. Li, Y. Cho, W. Yao, Y. Li, A. Cronk, R. Shimizu, M. A. Schroeder, Y. Fu, F. Zou, V. Battaglia, A. Manthiram, M. Zhang and Y. S. Meng, “Enabling high areal capacity for Co-free high voltage spinel materials in next-generation Li-ion batteries“, Journal of Power Sources 2020, 473, 228579
2. J. Alvarado, M. A. Schroeder, M. Zhang, O. Borodin, E. Gobrogge, M. Olguin, M. S. Ding, M. Gobet, S. Greenbaum, Y. S. Meng, K. Xu, “A carbonate-free, sulfone-based electrolyte for high-voltage Li-ion batteries” “supplementary data” Materials Today, 2018, 21(4), 341
The lithium metal subgroup focuses on the lithium metal anode fundamental science aiming to enable next-generation high-energy lithium metal batteries. The research area of the lithium metal subgroup includes but not limits to investigating the lithium metal failure mechanism from chemical, physical and mechanical perspectives, designing strategies to improve lithium metal performances and stability, including studying the reactivity of different electrolyte systems with Li and optimizing physical and electrochemical cycling parameters for Li metal anode. A variety of state-of-the-art characterization tools are utilized, including Titration Gas Chromatography, Cryogenic Electron Microscope, Cryogenic Focused Ion Beam, etc. The lithium metal subgroup currently has one postdoctoral researcher Wurigumula Bao and two Ph.D. students, Bingyu Lu and Diyi Cheng.
The cause and solution for the Li metal problems. The continuous formation of inactive Li is the direct cause of low CE, safety hazards and cell expansion in Li metal batteries. Liwhiskers with large tortuosity and heterogeneous SEI will facilitate the inactive Li formation and cause a series of problems; whereas if the deposited Li possesses a chunky morphology with minimal tortuosity and homogeneous SEI, the inactive Li formation will be significantly reduced, resulting in high CE.
Highlighted Publications:
1. C. Fang, J. Li, M. Zhang, Y. Zhang, F. Yang, J. Z. Lee, M. Lee, J. Alvarado, M. A. Schroeder, Y. Yang, B. Lu, N. Williams, M. Ceja, L. Yang, M. Cai, J. Gu, K. Xu, X. Wang & Y. S. Meng, “Quantifying inactive lithium in lithium metal batteries“, Nature, 2019, 572, 511–515
2. C. Fang, X. Wang, and Y. S. Meng, “Key Issues Hindering a Practical Lithium-Metal Anode” Trends in Chemistry, 2019, 1, 152
3. C. Fang, B. Lu, Y. S. Meng et al. “Pressure-tailored lithium deposition and dissolution in lithium metal batteries”, Under Review
4. B. Lu, Y. S. Meng et al. “Quantitatively Designing Porous Copper Current Collectors for Lithium Metal Anode”, Under Review
In many applications, energy density and low-temperature performance are two key metrics that state-of-the-art Li-ion batteries have significant difficulty meeting. In recent years, a lot of focus has been placed on the use of the Li-metal anode in combination with high voltage cathodes to dramatically increase the energy densities of batteries. Actual use of these systems has been hampered due to the unavailability of electrolytes that are compatible with the Li-metal anode while at the same time resistant to degradation at the high voltage cathode. Our liquefied gas electrolyte work focuses on gases that are promising candidates to achieve the stability required at both the anode and cathode in these aggressive, high voltage systems. At low temperatures or moderate pressures, these gases liquefy and can dissolve lithium salts to form liquefied gas electrolytes. These electrolytes have shown impressive compatibility with the Li-metal anode, and very good stability with high voltage cathodes as well as dramatically improved low temperature performance down to -60C.
Through experimental and computational approaches, our research has focused on the interesting solvation and transport mechanisms of the bulk electrolyte as well as the interfaces formed on both anodes and cathodes. Our work has opened a new area of research in the energy storage field and we hope to see new materials and manufacturing methods developed from the idea of using these gaseous solvents.
Highlighted Publications:
1. Y. Yang, Y. Yin, D. M. Davies, M. Zhang, M. Mayer, Y. Zhang, E. S. Sablina, S. Wang, J. Z. Lee, O. Borodin, C. S. Rustomji and Y. S. Meng, “Liquefied gas electrolytes for wide-temperature lithium metal batteries“, Energy Environ. Sci. 2020, 13, 2209 – 2219
2. Y. Yang, D. M. Davies, Y. Yin, O. Borodin, J. Z. Lee, C. Fang, M. Olguin, Y. Zhang, E. S. Sablina, X. Wang, C. S. Rustomji and Y. S. Meng, “High-Efficiency Lithium-Metal Anode Enabled by Liquefied Gas Electrolytes“, Joule , 3, 1–15, 2019
3. C. S. Rustomji, Y. Yang, T. K. Kim, J. Mac, Y. J. Kim, E. Caldwell, H. Chung, Y. S. Meng, “Liquefied Gas Electrolytes for Electrochemical Energy Storage Devices“, Science, 2017, 356, 1351
With the invention of non-aqueous electrolytes which enabled the use of alkali metal anodes, the energy density of batteries exhibited a remarkable improvement. However, in order to mitigate the safety issues the energy density was compromised and Li ion batteries (LIBs) were designed and got wide popularity especially in the potable electronic market. Fast approaching saturation curves of LIB performance matrices and pressing demands for higher energy and power density energy storage systems to support high energy demanding applications viz. electric vehicles make a situation inevitable to stretch out beyond LIBs. Revisiting Li metal anodes as in Li-O2 non-aqueous chemistry seems to be promising even though the understanding of the mechanism is in its infancy. Major bottle neck of exploiting the reversible reaction between Li+ and O2 is the sluggish kinetics of the electrochemical decomposition of the discharge product viz. Li2O2 to Li+ and O2 (Oxygen Evolution Reaction-OER). Fundamental understanding of Li-O2 electrochemistry is believed to pave way for other alkali Metal-Air batteries.
Various approaches aiming to improve the kinetic requirements of the fast charge/discharge include, addition of redox mediators, solubilizing agents etc. Carbonaceous matrices have been nanoengineered to facilitate the discharge process (Oxygen Reduction Reaction-ORR) and mass transport, thus currently playing pivotal role beyond just as catalyst support layer. Our recent oxyhalogen-sulfur electrochemistry approach synergistically combines these two and the round-trip efficiency is remarkably improved. Multi-faceted approaches to take control over the nucleation site, size and composition of the discharge products is critical in the design and fabrication of a practically usable Metal-Air battery.
Schematic depicting the mechanism of oxyhalogen-sulfur electrochemistry driven charge discharge processes
Highlighted Publications:
X. Wang, Y. Li, X. Bi, L. Ma, T. Wu, M. Sina, S. Wang, M. Zhang, J. Alvarado, B. Lu, A. Banerjee, K. Amine, J. Lu, and Y. S. Meng “Hybrid Li-Ion and Li-O2 Battery Enabled by Oxyhalogen-Sulfur Electrochemistry“, Joule. 2018, 2, 11, 2381
Research into inexpensive, long lifetime, grid energy storage options is increasingly important as we move to sustainable energy sources such as solar and wind. Sodium ion batteries are an exciting, inexpensive option for grid storage. The components of sodium ion batteries can be made with inexpensive elements such as manganese, iron, and carbon. While initial results show that sodium ion batteries have relatively high energy density, improvements in energy density and lifetime are necessary to make them a viable option.
To improve sodium ion batteries energy density and lifetime, we are studying the mechanism of energy storage in both the cathode and anode including material degradation and irreversible reactions. We use the many tools at our disposal, including novel synthesis methods and advanced characterization techniques. These advanced characterization techniques (XRD, TEM, FIB, NMR, synchrotron instruments, etc.) allow us to “see” what is happening inside the battery from atomic to macro scale. On the cathode side we are researching the irreversible reactions in high capacity inexpensive materials and how to increase the capacity by utilizing oxygen redox. On the anode side we are studying the irreversible reactions and how to increase their life time. We aim to determine the mechanism of energy storage in sodium ion batteries and use this knowledge to build better ones.
Highlighted Publications:
1. H. S. Hirsh, Y. Li, D. H. S. Tan, M. Zhang, E. Zhao and Y. S. Meng, “Sodium-Ion Batteries Paving the Way for Grid Energy Storage“, Adv. Energy Mater. 2020, 2001274
2. H. Hirsh, M. Olguin, H. Chung, Y. Li, S. Bai, D. Feng, D.Wang, M. Zhang and Y. S. Meng, “Meso-Structure Controlled Synthesis of Sodium Iron-Manganese Oxides Cathode for Low-Cost Na-Ion Batteries“, Journal of The Electrochemical Society, 2019, 166 (12) A2528
3. H. Li, H. Tang, C. Ma, Y. Bai, J. Alvarado, B. Radhakrishnan, S. P. Ong, F. Wu, Y. S. Meng, and C. Wu “Understanding the Electrochemical Mechanisms Induced by Gradient Mg2+ Distribution of Na-Rich Na3+xV2–xMgx(PO4)3_C for Sodium Ion Batteries” Chem. Mater., 2018, 30 (8), 2498
4. M. D. Radin, J. Alvarado, Y. S. Meng, and A. V. der Ven “Role of Crystal Symmetry in the Reversibility of Stacking-Sequence Changes in Layered Intercalation Electrodes”,Nano Letters, 2017, 17(12), 7789
5. J. Alvarado, C. Ma, S. Wang, K. Nguyen, M. Kodur, and Y. S. Meng, “Improvement of the Cathode Electrolyte Interphase on P2-Na2_3Ni1_3Mn2_3O2 by Atomic Layer Deposition“ACS Appl. Mater. Interfaces, 2017, 9(31), 26518
6. C. Ma, J. Alvarado, J. Xu, R. J. Clément, M. Kodur, W. Tong, C. P. Grey, and Y. S. Meng,”Exploring Oxygen Activity in the High Energy P2-Type Na0.78Ni0.23Mn0.69O2 Cathode Material for Na-Ion Batteries“, J. Am. Chem. Soc., 2017, 139(13), 4835
All-solid-state batteries (ASSB) offer high energy density and are a safer alternative to conventional liquid-electrolyte-based Li-ion batteries. However, electrolyte selection is limited, and those exhibiting competitive ionic conductivities often exhibit high interfacial impedance. Thin film batteries are ASSBs with thickness of only a few micrometers, which have the benefits of solid-state batteries with the potential to be incorporated in microelectronics. Further, they provide with ideal platforms for interfacial studies due to their well-defined geometries. Thin film subgroup in LESC has been exploring new thin film battery compositions and their interfaces, along which novel characterization techniques, including cryogenic focused ion beam (cryo-FIB), cryogenic scanning/transmission electron microscopy (cryo-S/TEM), solid-state nuclear magnetic resonance (ss-NMR) and neutron depth profiling (NDP), etc. have been incorporated to facilitate the interfacial study of air-/beam-sensitive battery materials.
Recently, we have demonstrated the structural and chemical evolution across the interphase between Li metal and LiPON via cryo S/TEM, which provides valuable insights for interface engineering to further improve the interfacial stability. Another work successfully resolved the structure of amorphous LiPON by ss-NMR coupled with DFT calculation, where LiPON is shown to be a low-connectivity glass, influencing its mechanical properties and its stability with Li metal. Future work of thin film subgroup will continue the study on the cathode/solid electrolyte interface along with developing cryogenic in situ methodologies for probing dynamic changes at the beam-sensitive interfaces and in phase-change materials.
Highlighted Publications:
1. D. Cheng, T. A. Wynn, X. Wang, S. Wang, M. Zhang, R. Shimizu, S. Bai, H. Nguyen, C. Fang, M. Kim, W. Li, B. Lu, S. J. Kim and Y. S. Meng, “Unveiling the Stable Nature of the Solid Electrolyte Interphase between Lithium Metal and LiPON via Cryogenic Electron Microscopy“, Joule, 2020, 4, 11, 2484-2500
2. M. A. T. Marple, T. A. Wynn, D. Cheng, R. Shimizu, H. E. Mason, and Y. S. Meng, “Local structure of glassy lithium phosphorus oxynitride thin films: a combined experimental and ab initio approach“, Angew. Chem. Int. Ed. 2020, 59, 2–11
3. J. Z. Lee, T. A. Wynn, M. A. Schroeder, J. Alvarado, X. Wang, K. Xu, and Y. S. Meng, “Cryogenic Focused Ion Beam Characterization of Lithium Metal Anodes“ ACS Energy Letters, 2019, 4, 489
4. J. Z. Lee, T. A. Wynn, Y. S. Meng, D. Santhanagopalan, “Focused Ion Beam Fabrication of LiPON-based Solid-state Lithium-ion Nanobatteries for In Situ Testing” . J. Vis. Exp, 2018, e56259
5. J. Z. Lee, Z. Wang, H. L. Xinb, T. A. Wynn, and Y. S. Meng, “Amorphous Lithium Lanthanum Titanate for Solid-State Microbatteries“, Journal of The Electrochemical Society, 2017, 164(1), 6268
6. Z. Wang, D. Santhanagopalan, W. Zhang, F. Wang, H. L. Xin, K. He, J. Li, N. Dudney, and Y. S. Meng,”In Situ STEM-EELS Observation of Nanoscale Interfacial Phenomena in All-Solid-State Batteries“, Nano Letters, 2016, 16 (6), 3760
Hybrid organic-inorganic perovskite materials, expressed as ABX3 where a monovalent cation (such as methylammonium (CH3NH3+), formamidinium (CH(NH2)2+), and Cesium (Cs+)) are located at A site, Sn or Pb is located at B site, and halide (I–/Br–/Cl–) is placed at X site, have been attained a lot of attention for their photovoltaic applications. Perovskite materials have great properties as high absorption coefficient, tunable bandgap, low exciton binding energy and long carrier diffusion length, thereby perovskite solar cells (PSCs) are now exhibiting greatly high power conversion efficiencies up to 24.2 % which is comparable performance to conventional polycrystalline Silicon photovoltaics. Though PSCs are close to Si photovoltaics, they still have some drawbacks as their humid/thermal instability, small scale and use of toxic Pb materials. Especially, long-term stability and large-area production should be achieved to take over Si photovoltaics role as a primary conventional solar cell.
So far, we achieved to elucidate the working mechanisms of hole transport materials and degradation process of PSCs. With the help of advanced characterizations like FIB-TEM, APT, and STEM-EELS, we were able to deeply understand their charge transfer and recombination mechanisms. Based on previous researches, we are now aiming for the commercialization of PSCs by scaling up and enhancing long-term stability of the PSCs. Device encapsulation or compositional engineering can provide better stability of PSCs and by developing efficient large-area producing method rather than spin-coating method, we can achieve large-area production of PSCs.
Highlighted Publications
1. M. Kim, S. Ham, D. Cheng, T. A. Wynn, H. S. Jung and Y. S. Meng, “Advanced Characterization Techniques for Overcoming Challenges of Perovskite Solar Cell Materials“, Adv. Energy Mater. 2020, 2001753
2. S. Wang, A. Cabreros, Y. Yang, A. S. Hall, S. Valenzuela, Y. Luo, J. Correa-Baena, M. Kim, Ø. Fjeldberg, D. P. Fenning and Y. S. Meng, “Impacts of the Hole Transport Layer Deposition Process on Buried Interfaces in Perovskite Solar Cells“, Cell Reports Physical Science, 2020, 1, 100103
3. S. Wang, Z. Huang, X. Wang, Y. Li, M. Günther, S. Valenzuela, P. Parikh, A. Cabreros, W. Xiong, and Y. S. Meng “Unveiling the Role of tBP−LiTFSI Complexes in Perovskite Solar Cells” J. Am. Chem. Soc 2018, 140 (48), 16720
4. S. Wang, M. Sina, P. Parikh, T. Uekert, B. Shahbazian, A. Devaraj, and Y. S. Meng, “Role of 4-tert-Butylpyridine as a Hole Transport Layer Morphological Controller in Perovskite Solar Cells” Nano Letters, 2016, 16, 5594
5. S. Wang, W. Yuan, and Y. S. Meng. “Spectrum-Dependent Spiro-OMeTAD Oxidization Mechanism in Perovskite Solar Cells“, ACS Appl. Mater. Interfaces, 2015, 7 (44), 24791
Fuel cells convert the chemical energy from fuels (such as hydrogen) to electrical energy. Solid oxide fuel cells (SOFCs) are one of the most attractive systems due to their high conversion efficiency; since they are electrochemical devices, their efficiency far surpasses combustion-based energy conversion. However, the operating temperature of current commercial SOFCs is around 800-950°C; at such temperatures, problems arise such as slow startup, reduced device durability, and limited material selection. To mitigate these issues, lower temperature operation is desired (550-700°C), and thus, interest has been growing in thin-film SOFCs (TF-SOFCs). By reducing the electrolyte layer thickness to a few micrometers, the electrolyte conductivity is maintained at lower temperatures and thus so is SOFC operation. Recently, we have developed a TF-SOFC (that contains a LSCF-YSZ cathode, GDC interlayer, YSZ electrode, and Ni-YSZ anode) fabricated completely by sputtering, in collaboration with Prof. Eric Fullerton’s group and Dr. Nguyen Q. Minh from Center for Energy Research. The cell demonstrated high power densities of 1.7 and 2.5 W/cm2 at 600 and 650°C, respectively.
(a) Schematic of TF-SOFC, (b) STEM cross-section image of the cell, and (c) magnified STEM image of the LSCF-YSZ cathode
For synthesis of the TF-SOFCs, the sputtering technique is utilized. Compared to atomic layer deposition (ALD), sputtering can enable control of the porosity of films by tuning the synthesis parameters. Pulsed laser deposition (PLD) is also a popular technique for ceramic-based film fabrication, but sputtering is superior in terms of cost and scalability. To characterize the SOFCs, along with electrochemical methods, we explore the morphology and chemistry in each layer of the cell through techniques such as scanning electron microscope (SEM, in conjunction with the focused ion beam (FIB)), (scanning) transmission electron microscope (TEM), and electron energy loss spectroscopy (EELS).
Highlighted Publications
1. Y. H. Lee, H. Ren, E. Wu, E. E. Fullerton, Y. S. Meng and N. Q. Minh, “All-Sputtered, Superior Power Density Thin-Film Solid Oxide Fuel Cells with a Novel Nanofibrous Ceramic Cathode“, Nano Lett. 2020, 20, 5, 2943–2949
2. H. Ren, Y. H. Lee, E. A. Wu, H. Chung, Y. S. Meng, E. E. Fullerton and N. Q. Minh, “Nano-Ceramic Cathodes via Co-sputtering of Gd−Ce Alloy and Lanthanum Strontium Cobaltite for Low-Temperature Thin-Film Solid Oxide Fuel Cells“, ACS Appl. Energy Mater. 2020, 3, 9, 8135–8142
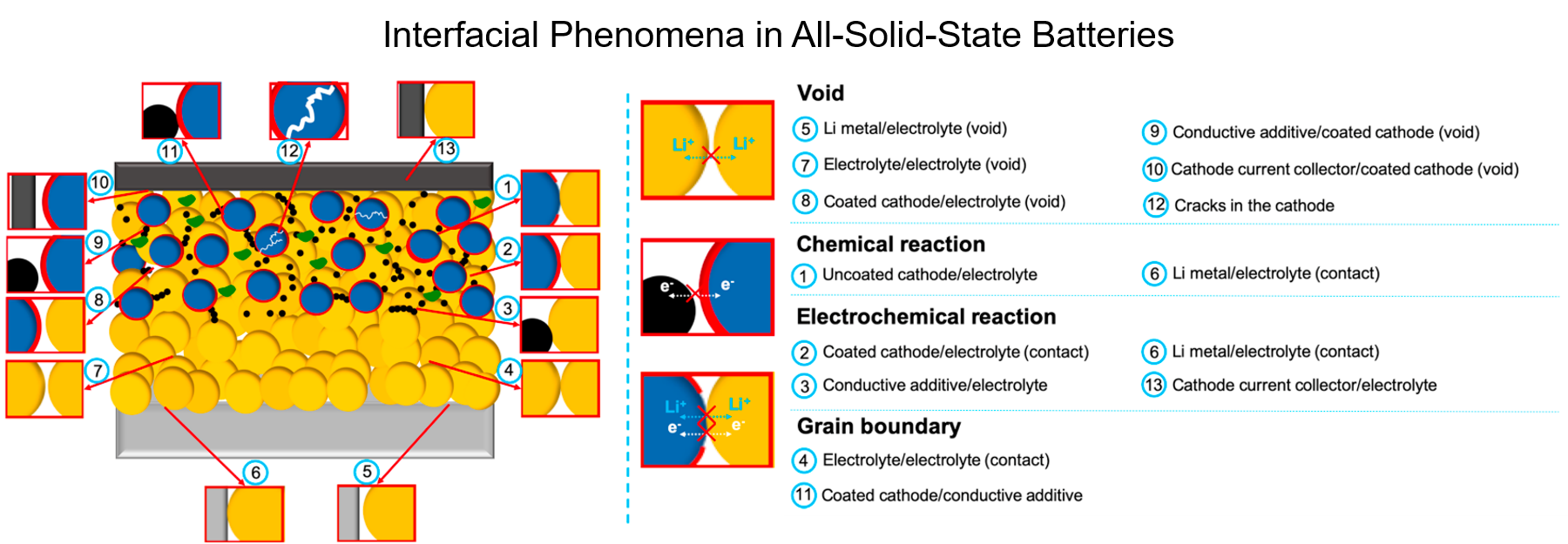
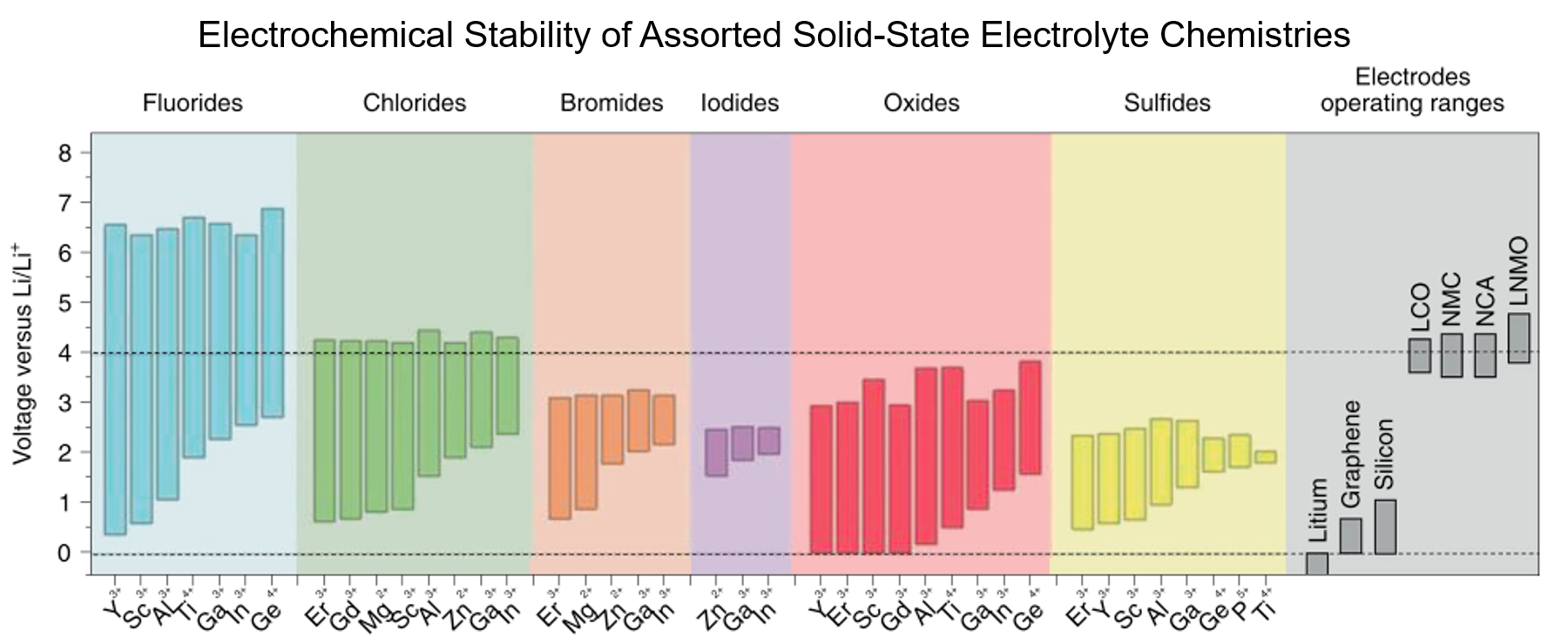
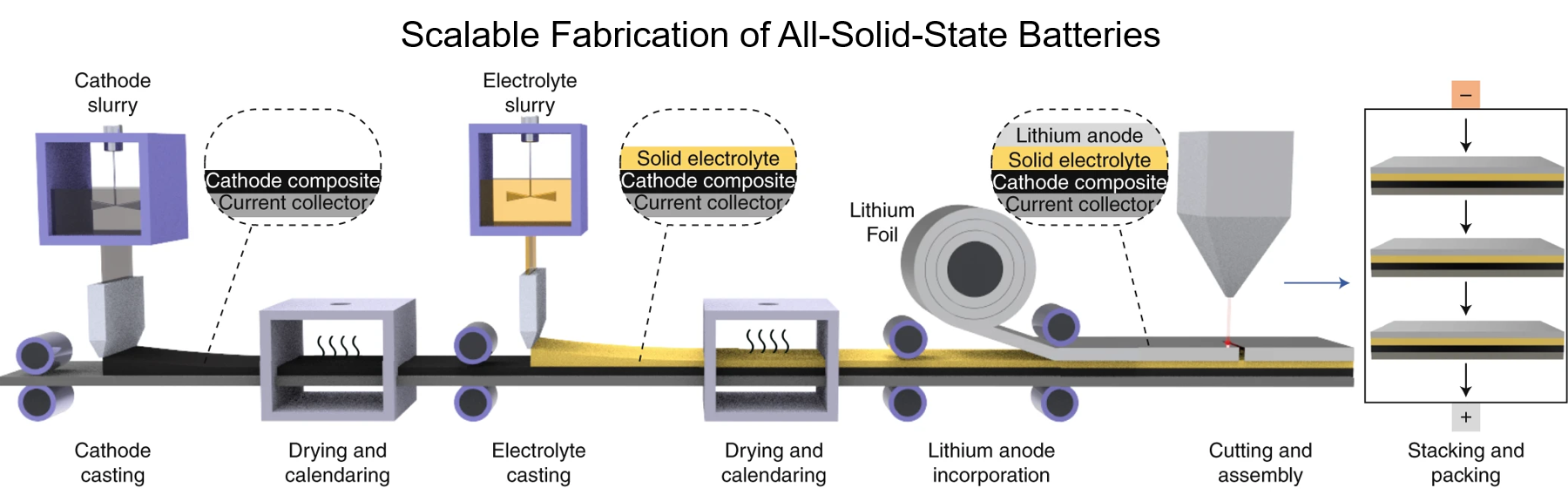
Highlighted Publications:
1. E. A. Wu, C. Jo, D. H. S. Tan, M. Zhang, J. Doux, Y. Chen, G. Deysher and Y. S. Meng, “A Facile, Dry-Processed Lithium Borate-Based Cathode Coating for Improved All-Solid-State Battery Performance“, Journal of The Electrochemical Society, 2020, 167, 130516
2. D. H. S. Tan, A. Banerjee, Z. Chen and Y. S. Meng, “From nanoscale interface characterization to sustainable energy storage using all-solid-state batteries“, Nature Nanotechnology, 2020, 15, 170–180
3. A. Banerjee, X. Wang, C. Fang, E. A. Wu and Y. S. Meng, “Interfaces and Interphases in All-Solid-State Batteries with Inorganic Solid Electrolytes“, Chem. Rev. 2020, 120, 14, 6878–6933
4. J. Doux, Y. Yang, D. H. S. Tan, H. Nguyen, E. A. Wu, X. Wang, A. Banerjeea, Y. S. Meng, “Pressure effects on sulfide electrolytes for all solidstate batteries“, J. Mater. Chem. A, 2020, 8, 5049–5055
5. J. Doux, H. Nguyen, D. H. S. Tan, A. Banerjee, X. Wang, E. A. Wu, C. Jo, H. Yang and Y. S. Meng, “Stack Pressure Considerations for Room-Temperature All-Solid-State Lithium Metal Batteries“, Adv. Energy Mater. 10, 1903253, 2019
6. H. Nguyen, A. Banerjee, X. Wang, D. Tan, E. A. Wu, J. Doux, R. Stephens, G. Verbist, Y. S. Meng, “Single-step synthesis of highly conductive Na3PS4 solid electrolyte for sodium all solid-state batteries“, Journal of Power Sources, 435, 126623, 2019
7. D. H. S. Tan, E. A Wu, H. Nguyen, Z. Chen, M. A. T. Marple, J. Doux, X. Wang, H. Yang, A. Banerjee and Y. S. Meng, “Elucidating Reversible Electrochemical Redox of Li6PS5Cl Solid Electrolyte“, ACS Energy Lett. 4, 10, 2418−2427, 2019
Silicon anode subgroup is involved towards developing the next generation of alloy anodes to replace graphite, for increased cell energy density. Silicon anodes can provide high theoretical specific capacity (~4000 mAh/g) but suffer from large volume expansion (~300%) and subsequent contraction during lithiation and delithiation, that severely affects its capacity retention. Our efforts are geared towards solving this fundamental issue through binder engineering, pre-lithiation strategies and exploring silicon oxide as an alternative solution for stable capacity retention. Our team explores the realm of both half-cell and full cell configurations to understand the effects that arise from both the anode and cathode.
Our expertise in diagnosing battery materials through advanced characterization, using tomography for 3-D visualization, microscopy and spectroscopy (TEM, XPS) and electrochemistry provides us with a deeper understanding of the issues and help us to provide more targeted and effective solution to current challenges.
Highlighted Publications:
1. P. Parikh, M. Sina, A. Banerjee, X. Wang, M. Savio D’Souza, J.-M. Doux, E. A. Wu, O. Y. Trieu, Y. Gong, Q. Zhou, K. Snyder, and Y. S. Meng, “Role of Polyacrylic Acid (PAA) Binder on the Solid Electrolyte Interphase in Silicon Anodes” Chemistry of Materials, 2019, 31 (7), 2535
2. H. Shobukawa, J. Alvarado, Y. Yang, Y. S. Meng, “Electrochemical performance and interfacial investigation on Si composite anode for lithium ion batteries in full cell”, Journal of Power Sources, 2017, 359, 173
3. M. Sina, J. Alvarado, H. Shobukawa, C. Alexander, V. Manichev, L. Feldman, T. Gustafsson, K. Stevenson, Y. S. Meng,”Direct Visualization of the Solid Electrolyte Interphase and Its Effects on Silicon Electrochemical Performance” Adv. Mater. Interfaces 2016, 1600438
4. H. Shobukawa, J.W. Shin, J. Alvarado, C. S. Rustomji and Y. S. Meng, “Electrochemical reaction and surface chemistry for performance enhancement of a Si composite anode using a bis(fluorosulfonyl)imide-based ionic liquid”, Journal of Materials Chemistry A., 2016, 4, 15117
Attributed as the first practical battery developed, Zinc batteries have the known benefits of low material cost, non-toxicity, and a high theoretical energy density. Zinc-based batteries are commercially implemented in the medical and stretchable electronics industry and have great promise in grid storage. In our lab, we focus on high throughput, printable, low cost, and energy dense batteries with superior power densities when powering Bluetooth and wireless devices. Shown below from our group’s recent publication, with our all-printed and stacked configuration, our Zinc-based batteries show superior areal capacities and can handle higher current densities than reported in literature. These performances are best illustrated from the images and video below in which our printed Zinc batteries power the high current pulse required of a Bluetooth enabled flexible display.
The development of this high-performance battery is largely due to our arsenal of advance characterization techniques that allow us to recognize failure modes and optimize our materials design. Shown below is an example of how micro-scale X-ray Computed Tomography (micro-CT), used in our work, can shed light on our battery’s structure, in which each layer can be non-destructively probed to obtain 3D reconstructions. Future work will showcase improved performances through our advance characterization and will shed light on how such techniques can aid the battery community for the future of high performance and low-cost batteries.
Highlighted Publications:
1. L. Yin, J. Scharf, J. Ma, J. Doux, C. Redquest, V. L. Le, Y. Yin, J. Ortega, X. Wei, J. Wang and Y. S. Meng, “High Performance Printed AgO-Zn Rechargeable Battery for Flexible Electronics“, Joule, 2020, 5, 1-21 [Video content: YouTube Link]
2. R. Kumar, J. Shin, L. Yin, J.-M. You, Y. S. Meng, Joseph J Wang, “All-Printed, Stretchable Zn-Ag2O Rechargeable Battery via, Hyperelastic Binder for Self-Powering Wearable Electronics“, Adv. Energy Materials, 2017, 1602096
3. A. Bandodkar, W. Jia, J. Ramirez, Y. S. Meng and J. Wang, “An epidermal alkaline rechargeable Ag-Zn printable tattoo battery for wearable electronics”, J. Mater. Chem. A, 2014, 2(38), 15788
4. J.W. Shin, J.-M. You, J. Z. Lee, R. Kumar, L. Yin, J. Wang, and Y. S. Meng,”Deposition of ZnO on bismuth species towards a rechargeable Zn-based aqueous battery” , Phys. Chem. Chem. Phys., 2016, 18, 26376 (Front Cover)
The Computational Materials Science Group at LESC uses atomistic scale modeling to provide predictive understanding of Li-ion battery materials physicochemical properties.
We employ highly accurate first-principles and classical molecular dynamics techniques to study the electronic structure and energetics of anode, bulk electrolyte, cathode, and interface systems on massively parallel supercomputers. These computational chemistry approaches are performed in close collaboration with experimentalists via a complimentary research strategy with the aim of designing functional state-of-the-art battery materials.
Highlighted Publications:
1. Y. S. Meng, M. E. A.-d. Dompablo, (invited review) “First principles computational materials design for energy storage materials in lithium ion batteries“, Energy & Environmental Science, 2009, 2, 589
2. B. Xu, C. R. Fell, M. Chi, and Y. S. Meng, “Identifying surface structural changes in layered Li-excess nickel manganese oxides in high voltage lithium ion batteries: A joint experimental and theoretical study“, Energy & Environmental Science, 2011, 4, 2223
3. T. A. Wynn, C. Fang, M. Zhang, H. Liu, D. M Davies, X. Wang, D. Lau, J. Z Lee, B.-Y. Huang, K. Z. Fung, C.-T. Ni and Y. S. Meng “Mitigating Oxygen Release in Anionic-Redox-Active Cathode Materials by Cationic Substitution through Rational Design“, JMCA 2018, 6, 24651
4. I.-H. Chu, M. Zhang, S. P. Ong, and Y. S. Meng, “Handbook of Materials Modeling-Battery Electrodes, Electrolytes, and Their Interfaces“